The Performance Enhancing Phenomenon You Didn’t Know About
- Linked Fit
- Aug 10, 2022
- 9 min read

Post-Activation Potentiation (PAP) is a physiological phenomenon that increases the rate of force development (e.g. the ability to produce force quickly). Therefore, PAP is most commonly used to enhance power performance (e.g. an activity involving quick force executions such as a vertical jump). PAP is traditionally achieved by completing a heavy resistance exercise (i.e., the conditioning or preloading event) before a biomechanically similar explosive exercise (i.e., a short sprint or jump). A typical example includes completing a heavy barbell back squat before a vertical jump. Enhanced power performance during the vertical jump may be recognized through improved jump height, ground-reaction force, or take-off velocity.
This article will discuss how PAP can be achieved and the underlying physiology of PAP. Lastly, how PAP can be applied to improve athletic and/or training performance measures.
Evidence of PAP
Research on PAP has noted performance enhancements in the upper and lower body!
Before diving into the mechanisms of PAP, it is noteworthy to recognize some current research. PAP has been extensively studied in exercise science, producing many instances where PAP has been implemented to produce performance benefits. Research on PAP has noted performance enhancements in the upper and lower body. Additionally, it has progressed to better define PAP by detecting underlying physiology, determining approximate methodologies to induce it, and determining how long performance enhancements last. Further research is still needed on alternate methods to induce PAP beyond the traditional heavy load and how the PAP effect can be optimized based on individual differences.
Improved upper body performance was found by Baker (2003). Baker produced a 4.5% increase in power output during explosive bench press throws after completing 6-repetitions of a bench press at 65% of one’s 1-repetition maximum (RM) (2003). Additionally, in a study by Sas-Nowosielski & Kandzia, 5RM pull-ups caused a 6.8% improvement in the power performance of climbers (2020).
In terms of lower body performance improvements, Gourgoulis et. al. (2003) found a 2.39% improvement in vertical jump performance following five sets of half squats at increasing intensities. Moreover, Weber et. al. (2008) produced a 4% increase in vertical jump height following back squats with 85% 1RM.
Although the percent increases in power performance may seem small, they are significant performance improvements by research statistical analysis. Additionally, these improvements are immediate since they are achieved within minutes. Therefore, by utilizing the PAP phenomenon, one can improve performance levels beyond their potential maximum capabilities from consistent training adaptations. Athletes can reach new optimal performance levels when used before an athletic performance measure. Differences in PAP achievement and percentage of performance improvement can be attributed to differences in the methods in each study. There are many considerations when developing a protocol to induce PAP. These considerations will be discussed further in the following section.
Traditional Elicitation of PAP
Intensity, volume, and rest period determine the effectiveness of a preloading event in producing subsequent performance enhancement.
PAP onset and following performance gains depend on the intensity and volume of the conditioning activity and the rest period between the conditioning activity and the power performance. Research on PAP has considered multiple loads, sets, repetitions, and rest periods.
Studies suggest that the greatest PAP effects are achieved after completing a conditioning activity with a load of 80% or greater of one’s 1 RM (Baker, 2003; Tillin & Bishop, 2009; Villalon-Gasch et. al., 2020). Additionally, the conditioning activity should be completed for 1-3 sets with 1-5 repetitions (Villalon-Gasch et. al., 2020). A prolonged preloading event of greater intensity is believed to exploit PAP to a greater effect (Sale, 2002). However, in this case, as PAP increases, so does fatigue. Fatigue diminishes performance enhancements of PAP. Therefore, performance enhancement is greatest when there is a balance between PAP and fatigue.
To achieve the optimal balance between PAP and fatigue, there should be a rest period between the conditioning activity and the performance. Since fatigue dissipates faster than PAP, the rest period allows the PAP effect to overcome fatigue (Tillin & Bishop, 2009). A rest period between 2-10 minutes supports the greatest PAP performance enhancements (Tillin & Bishop, 2009). The rest period should be closer to 10 minutes with a more intense conditioning activity since more fatigue has developed, which masks the PAP effects. On the other hand, with a less intense conditioning activity, the rest period should be shorter since less fatigue occurs and the PAP effect may be less. With recovery, although fatigue diminishes faster, PAP also decreases. Consequently, the rest period mustn't be long enough to decay the entirety of the PAP mechanism, causing a loss of performance benefits.
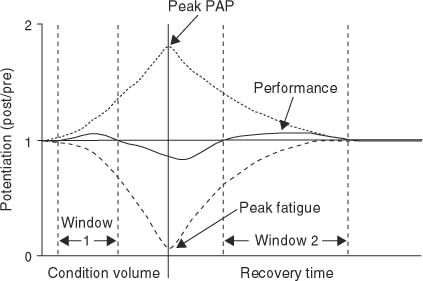
Relationship between PAP and Fatigue. With low conditioning volume (i.e., 1-repetition of 90% 1RM back squat), PAP is greater than fatigue, and following performance enhancements can be executed almost immediately in window 1. With increasing conditioning volume (i.e., 6 repetitions at 80% 1RM), fatigue dominates the PAP effects. A rest period is needed for fatigue to diminish; the effect during the subsequent performance is evident in window 2. (Tillin & Bishop 2009).
Physiological Mechanism of PAP
PAP is proposed to be a result of phosphorylation of myosin regulatory light chains and increased recruitment of type II muscle fibers.
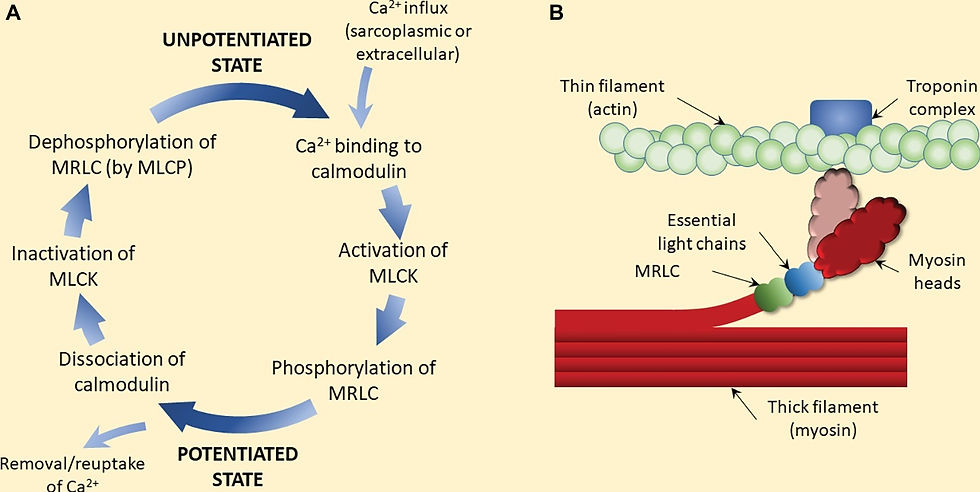
When completing a preloading task to induce PAP, an activated state is established within the worked muscles. Research suggests that this activated state is explained by the physiological processes triggered by the conditioning activity (Hodgson, 2005; Sale, 2002; Tillin & Bishop, 2009). One such process is the phosphorylation of myosin regulatory light chains (Tillin & Bishop, 2009). Muscles contain contractile components called sarcomeres, made up of thick (e.g. myosin) and thin (e.g. actin) filaments. During a muscle contraction, regulatory proteins react with calcium to expose binding sites on the myosin head (e.g. end of the filament) that cause myosin and actin to bind and form cross-bridges. When this happens, the sarcomere shortens, initiating a muscle contraction. Regulatory light chains are positioned at the neck of the myosin head (Tillin & Bishop, 2009). When a phosphate molecule binds to these chains (e.g. phosphorylation), the structure of the myosin head is altered, and its ability to bind to actin is more rapid, thus increasing the strength of subsequent contractions (Hogson, 2005; Tillin & Bishop, 2009). Additionally, phosphorylation of regulatory light myosin chains makes the myosin-actin complex more sensitive to calcium, facilitating muscle contraction.
The second physiological process underlying PAP is increased recruitment of type II muscle fibers (Tillin & Bishop, 2009). Muscle fibers consist of slow twitch (e.g. type I) and fast twitch (e.g. type II) muscle fibers. Type II muscle fibers are considered fast twitch because they can produce larger amounts of force in little time and support short-duration activity. Therefore, these fibers are critical in strength and power movements where it is required that force is produced quickly. A motor unit consists of a motor neuron and all muscle fibers it innervates. For the muscle fibers to contract, the motor neuron must emit acetylcholine, a neurotransmitter that tells the muscle fibers to contract. More muscle fibers are activated when more motor units are recruited, and greater force can be produced. A preloading event decreases the likelihood that a neurotransmitter will fail when crossing the junctions between neurons, increasing the recruitment of higher order motor units and type II muscle fibers (Tillin & Bishop, 2009). Furthermore, phosphorylation of myosin light regulatory chains is greater in type II muscle fibers (Sale, 2002). These two factors result in the ability to produce greater amounts of power.
Application of PAP
Through preloading and complex training, PAP can be utilized for performance enhancement.
There is evidence that PAP can provide short-term performance enhancement for athletes. For PAP to be utilized this way, traditional elicitation methods could be incorporated into a warm-up before a performance, leading to improvements in sprinting and jumping ability (Robbins, 2005; Sale, 2002). Considering the equipment requirements to achieve heavy loads and induce PAP through traditional means, alternative methods have also been proposed. It is possible that the use of blood flow restriction during a resistance exercise can simulate a high load to elicit PAP (Doma et. al., 2020). However, more research is needed for both methods on training variables such as intensity, sets, repetitions, and rest period to determine what is optimal for athletes.
Furthermore, elicitation of PAP varies depending on individual differences such as muscular strength, training level, muscle fiber type distribution, and strength-power ratio (Tillin & Bishop, 2009). It is proposed that stronger, higher trained athletes experience more benefits from PAP (Robbins, 2005). Therefore, the PAP protocol may need to be individualized.
It is also possible that through complex training, PAP can be manipulated to lead to long-term neuromuscular changes. Complex training involves pairing a heavy resistance exercise with a power exercise (e.g. plyometrics) that is biomechanically similar within the same set (NSCA, 2016). Complex training includes pairing a back squat with a box jump to develop lower body power or a bench press with a med ball chest pass to develop upper body power. Training during the power exercise is enhanced due to the activated state created by the heavy preloading exercise, allowing the athlete to train at a higher level (Robbins, 2005). Complex training has been shown to cause positive neuromuscular adaptations (Cormier et. al., 2020). Moreover, the contrasting exercises, heavy resistance exercise (e.g. low velocity, high load) and explosive plyometric (e.g.high velocity, low load), stimulate both ends of the force-velocity relationship (Cormier et. al., 2020). For this reason, it is theorized that complex training could be more effective than resistance or plyometric training alone in developing power production capabilities (Cormier et. al., 2020; Robbins, 2005).
Examples of how LF utilizes PAP in programming:
Push - Horizontal: Improving Bench Press performance
Perform three repetitions of this movement.
Perform the programmed amount of repetitions.
Squat: Improving Squat performance
Perform three repetitions of this movement.
Perform the programmed amount of repetitions.
Pull - Vertical: Improving Pull Up performance
Perform three repetitions of this movement.
Perform the programmed amount of repetitions.
PAP For the Common Recreationally Active Individual
Although PAP research aims to improve athletic performance, PAP benefits have been recognized in recreationally active individuals (De Freitas et. al., 2021; Gourgoulis et. al., 2003). Similar to athletes, these benefits have been elicited in traditional means to enhance power performance (Gourgoulis et. al., 2003). However, recreationally active individuals may experience more fatigue after acute heavy resistance exercise (Chui et. al., 2003). Therefore, the PAP protocol may need to differ slightly by decreasing intensity or including a longer rest period for potentiation to overcome fatigue and allow PAP benefits to be experienced.
Since many athletic sports require great power performance, eliciting PAP to enhance power performance is ideal for the PAP phenomenon. Yet, traditionally, using the PAP phenomenon may not be as common for recreationally active individuals because increasing jumping and sprinting abilities is not desirable. The PAP effect can also be utilized to improve everyday weight room performance. Dynamic stretching of target muscles has been shown to heighten neuromuscular activity and improve exercise performance (Hough et. al., 2009). Moreover, the PAP phenomenon could improve muscle hypertrophy in trained individuals through low repetitions of heavy resistance as a preloading event to achieve greater repetitions during moderate load exercise (De Freitas et. al., 2021).
Conclusion
In conclusion, the PAP effect occurs after muscles are primed from a conditioning activity due to increased phosphorylation of myosin regulatory light chains and improved motor unit recruitment. The PAP elicitation protocol must be manipulated to ensure potentiation overcomes fatigue to realize benefits such as increased force production and power output. Athletes can use the PAP phenomenon to enhance jumping and sprinting above normal training adaptations immediately before a performance. Recreationally trained individuals can also utilize the PAP phenomenon to improve weight room performance or achieve personal goals. Still, more research is needed on how individual differences affect the optimal protocol for achieving PAP.

References
Baker, D., (2003). Acute effect of alternating heavy and light resistances on power output during upper-body complex power training. Journal of Strength and Conditioning Research, 17(3), 493–497.
Chiu, L. Z., Fry, A. C., Weiss, L. W., Schilling, B. K., Brown, L. E., & Smith, S. L. (2003). Postactivation potentiation response in athletic and recreationally trained individuals. The Journal of Strength & Conditioning Research, 17(4), 671-677.
Cormier, P., Freitas, T. T., Rubio-Arias, J. Á., Alcaraz, P. E., (2020). Complex and contrast training: Does Strength and Power Training Sequence Affect Performance-Based Adaptations in Team Sports? A Systematic Review and Meta-analysis. Journal of Strength and Conditioning Research, 34(5), 1461-1479.
De Freitas, M. C., Rossi, F. E., Colognesi, L. A., De Oliveira, J. V. N., Zanchi, N. E., Lira, F. S., ... & Gobbo, L. A. (2021). Postactivation potentiation improves acute resistance exercise performance and muscular force in trained men. The Journal of Strength & Conditioning Research, 35(5), 1357-1363.
Doma, K., Leicht, A. S., Boullosa, D., Woods, C. T., (2020). Lunge exercises with blood-flow restriction induces post-activation potentiation and improves vertical jump performance. European Journal of Applied Physiology, 120, 687-695.
Gourgoulis, Aggeloussis, N., Kasimatis, P., Mavromatis, G., & Garas, A. (2003). Effect of a submaximal half-squats warm-up program on vertical jumping ability. Journal of Strength and Conditioning Research, 17(2), 342–344.
Hodgson, M., Docherty, D., & Robbins, D. (2005). Post-activation potentiation. Sports Medicine, 35(7), 585–595.
Hough, P. A., Ross, E. Z., & Howatson, G. (2009). Effects of dynamic and static stretching on vertical jump performance and electromyographic activity. The Journal of Strength & Conditioning Research, 23(2), 507-512.
National Strength and Conditioning Association [NSCA]. (2016). Essentials of strength training and conditioning. (G. Haff & N. T. Triplett, Eds.). Human Kinetics
Sas-Nowosielski, & Kandzia, K. (2020). Post-activation Potentiation Response of Climbers Performing the Upper Body Power Exercise. Frontiers in Psychology, 11, 467–467.
Sale, D. G., (2002). Postactivation potentiation: Role in human performance. Exerc. Sport Sci. Rev., 30(3), 138-143.
Tillin, N. A., & Bishop, D., (2009). Factors modulating post-activation potentiation and its effect on performance of subsequent explosive activities. Sports Medicine, 39(2), 146-166.
Villalon-Gasch, L., Jimenez-Olmedo, J. M., Sebastia-Amat, S., & Pueo, B., (2020). Squat base post-activation potentiation improves vertical jump of elite female volleyball players. Journal of Physical Education and Sport, 20(4), 1950-1956.
What Benefits Do Press Release Distribution Services Offer Businesses?
Press release distribution services provide numerous benefits to businesses, making them a vital tool for growth and visibility. They enhance brand awareness by ensuring press releases are seen by targeted audiences, including journalists, bloggers, and potential customers. Services like Pr Wires, known for offering the best PR distribution service, help businesses secure valuable media coverage, build credibility, and increase their online presence. These services also improve SEO rankings by generating backlinks, boosting website traffic, and ensuring content reaches authoritative sources. Additionally, they provide measurable results, allowing businesses to track the impact of their press releases and refine future strategies.
Where to Find Assignment Help for NYU Courses Online?
Finding reliable assignment help for NYU courses online can make a huge difference in academic success. Students often look for platforms that understand the demands of NYU's curriculum. One trusted name is BookMyEssay, offering expert guidance and tailored solutions. In the middle of this academic journey, their new classes NYU Assignment Help online provides timely support, especially for complex subjects and tight deadlines. BookMyEssay ensures that NYU students receive well-structured, plagiarism-free content that aligns with university standards. With access to expert writers and 24/7 support, students can confidently tackle challenging coursework and improve their performance without unnecessary stress or delays.